As the global population is still rising – albeit at a decreasing rate – we can expect an additional billion or more people in the next 10 years and yet another billion by 20401. Simple mathematical calculations would suggest that the number of off-grid global poor will nearly double within a decade. And the world is also urbanising quite rapidly, thus a considerable proportion of the marginal poor can be expected to relocate to densely populated near-urban off-grid areas.
Successful off-grid energy solutions, however, can promote migration into developing rural communities, thereby giving them semi-urban characteristics. The growth of a semi-urban sector should not, however, be a cause for concern: in fact it can act as a buffer against the unsustainable growth of mega-cities with expanding slums, and point towards more sustainable models. In addition, the availability of off-grid electrical power for decent lighting, pumping, refrigeration, sanitation, education, communication, audio-visual and entertainment facilities, will enhance rural life and development2.
Current position
The off-grid energy paradigm for electrification and development of the global rural population must be closely associated with the evolution of clean, green and low-carbon power. It is heartening to note the many ongoing and forward-looking rural projects such as Lighting Africa, the Pico-PV Programme, Husk Power Systems, the Jawaharlal Nehru National Solar Mission and Lighting India, to name just a few. Millions of poor people in the countryside now have access to electricity from photovoltaic (PV) modules alongside storage batteries that can generate 12, 48 or even higher voltages to run household and community appliances.
Photovoltaic systems are favourable options for meeting low energy demand – of less than 5 kilowatt hours per day – at steady loads in remote locations that regularly receive good solar flux. Solar lighting based on light-emitting diode (LED) lamps and solar lanterns are not only clean, efficient and reliable, but are cost effective in competition with kerosene lamps. There are also examples of rural electrification where engine-generators – diesel-driven gensets – are used for heavier loads along with PV systems in hybrid configurations.
An excellent model of micro-grid development is Mera Gao Power, an enterprise operating in partnership with USAID, which is able to build, own and operate micro-grids in rural communities in India for costs reported to be below US$ 1,000 per village. In each village setting they provide a centrally located solar panel combined with a bank of lead-acid batteries for storing and then delivering energy when solar flux is not available3. Within a period of two years, Mera Gao has been able to provide electricity to 500 off-grid villages, providing 65,000 people with lighting and mobile-phone charging facilities.
Hydropower in mini-grids has been implemented in a few rural locations with access to flowing water. Small wind turbines dependent on regular wind speeds higher than 15 kilometres per hour have been installed in some locations for charging batteries, and turbines can also lend themselves to larger infrastructure such as community-level local grids. However, as renewable sources such as solar and wind power tend to be intermittent, energy availability can be sporadic without the use of battery packs.
While biomass – mainly wood – has been an important source of energy for cooking in rural communities for centuries, the new opportunity to generate combined heat and power using generators has become a growing reality4. Several gasifiers have been made available within rural communities, where they are run on locally available biomass such as rice husks, wood chips, lentil or cotton stalks and other agro-residues including animal and kitchen wastes. These gasifiers can power generators to provide heat and power for a community or hamlet of typically 1,000–2,000 people. In another pioneering example in Uganda, it is reported that maize mill owners produce and sell power to households via mini-grids or battery charging.
New opportunities
Solar energy is expected to contribute more than 10 per cent of the energy mix in large developing countries and regions by 2022, driven by the goal of providing electricity to the hundreds of millions of rural people who do not have it now. There is a great opportunity to skip highly polluting forms of energy and move straight to cleaner sources such as solar power. Leapfrogging in this way will avoid the large costs of transmission lines while benefiting the environment and the health of the people. Off-grid electrification is an innovation that should be harnessed for entrepreneurship and gainful employment. In addition, the very villages that are expected to be the subject of this e-transformation are also the source of a demographic dividend arising from a youthful population.
Energy storage
Renewable energy technologies are not stand-alone solutions in the sense that solar PV and wind-based systems require batteries for storage along with inverters to convert direct current to alternating current, and battery chargers; gensets require a regular supply and storage of diesel or petrol; and hydropower, while relatively economic to operate, is dependent on the availability of fast-moving water throughout the year.
Technologies have evolved over many decades that allow us to convert the energy from sunlight into electricity through the PV cell, but this then needs to be stored in batteries and made available without interruption over a number of days. Battery packs based on lead-acid batteries are already used in rural off-grid electrification schemes for storing energy from renewable sources during peak production – and are also in demand in rural areas as a direct source of electricity for lighting or phone charging.
Lead-acid batteries currently provide the most economical electrical storage technology. Typically, these are designed for deep-discharge cycles that provide a small current for many hours between two charge cycles, using up most of the stored energy. Batteries store their energy as chemical energy in two separated electrodes. The separated chemicals can then be made to react via an electrolyte, feeding back the chemical energy as electrical energy.
Batteries can be charged and discharged over hundreds or even thousands of cycles, after which they become spent and cannot be used again. Given that the discharge of lead into the environment is not an option due to its toxicity, batteries must be recovered and recycled to make new ones. The good news is that lead is not difficult to recycle: in fact in developed economies and in the urban space of rapidly emerging economies, the infrastructure for collecting, dismantling and recycling batteries is very successful.
Recycling
Mature technology for recycling lead batteries is based on heavy investment in large high-temperature furnaces backed by environmental control of discharged dust, water and gases. Such relatively expensive and large-scale technologies are currently outside the practical realm of the rural sector. Nevertheless, there is a thriving – though often poorly regulated or even unauthorised – rural sector based on small-scale units and driven by the economic incentive of the resale value of lead. Unfortunately, however, small-scale units are unable to melt all of the lead compounds in the spent batteries, creating a massive potential health burden if unrecovered lead compounds make their way into the local environment.
Where growth in rural off-grid electrification through renewables is envisaged, as in smart villages, the demand for back-up storage batteries will intensify. A new technology for safely recovering lead from batteries in small-scale units has recently been developed at the University of Cambridge (Figure 1), precisely in anticipation of growth in battery usage spurred by rural electrification through renewables5. This battery technology can also be used in electric scooters, whose growth is anticipated as rural development progresses further. It is possible to imagine that, in the near future, more than 95 per cent of all lead – more than 20 million tonnes per year by 2022 – will be used in vehicle batteries and for back-up and emergency power supply, and most of this will be supplied by recovering lead from used batteries. An environmentally sustainable process that can be operated on a small scale – but also a large one – is already in demand and will further expand. The rural sector can lead this new technology.
Since economy of scale is not a limiting factor, such a technology will provide an opportunity for nucleating and retrofitting many rural, smaller-scale lead-battery industries in rapidly growing economies. This new green cottage industry will have a major positive economic impact for a large number of people dependent on lead batteries for their livelihood and for the development of their local economies.
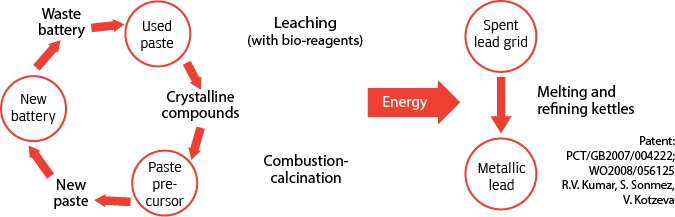
Residual battery paste is dissolved in an aqueous solution of carboxylic acids derived from
plants (leaching) to produce lead organic material which is converted by combustion at high
temperatures to lead monoxide and metallic lead for new battery paste preparation. The
lead grids are refined separately using heat from the first process. The use of acids from
plants results in a very low carbon footprint. Thus it is possible for rural users to send back
to battery manufacturers value-added products for re-engineering.
Can fuel cells play a role in the future?
A fuel cell can generate electricity silently and without combustion. The fuel stream is fed into a compartment separated from a second compartment into which air is fed. The two streams never mix or burn, but still electricity is produced at efficiencies that can be more than 100 per cent higher than turbine-based power plants using the same fuel. In principle,the fuel cells are similar to batteries. The secret material in both cases is the separator – an electrolyte – which allows for silent but active communication between the fuel and the air (Figure 2).
Fuel cells operating at high temperatures can also generate heat along with electricity, thus making them uniquely placed for heat-and-power solutions. A well designed combined heat-and-power unit can harness heat that would otherwise be wasted, transferring it to a heating fluid that can be used as a hot-water storage system.
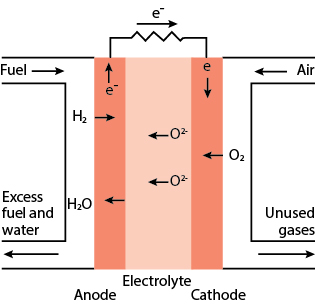
An ideal fuel of the future is hydrogen, its main advantage being that the only waste product of using it for electricity generation is water. We are not ready for a local hydrogen economy as yet, and the storage and transportation of hydrogen is still a developing technology. But increasing use of the fuel cell as an off-grid technology will result in a short lead-time for adopting hydrogen fuel cells in the future.
If hydrogen is not an option for now, what will the fuel cell be in a rural setting? Biomass gasifiers using agricultural waste are already in use in the rural sector for generating power using conventional turbine technology. Fuels produced from biomass gasifiers contain hydrogen and carbon monoxide, which can be directly fed to the fuel cells and efficiently converted to electricity. The NonFerrous Materials Technology Development Centre (NFTDC) based in Hyderabad, India, in collaboration with the University of Cambridge, UK, is currently developing a low-cost fuel-cell stack suitable for rural application6.
Both the gasifiers and the fuel-cell stacks lend themselves to micro-grid operation and provide incentives for community action, participation, employment and entrepreneurship. It is possible either to distribute the fuel to households with small fuel-cell stacks for individual electricity generation or to produce electricity at a community/hamlet level for distribution to households.
Biomass is a carbon-neutral source of power, as it embodies nature’s living carbon within renewable stock and does not imperil the health of the carbon cycle – in contrast with the “dead carbon” in fossil fuels. A biomass-based fuel using local wastes for generating electricity through a fuel cell is a good model for moving up the energy ladder.
Conclusions
The fact that the electricity sector is quietly undergoing a globally disruptive transformation offers new opportunities for tackling electricity poverty. Rapid expansion of zero-emission solar power backed by energy storage in batteries is set to take off, both within the grid infrastructure and off the grid. Some estimates forecast 200 gigawatts of battery-backed solar power by 2025, a fourfold increase on 2015 – and a fortyfold increase on 2005. At the heart of this transformation is massive cost reduction in both solar cells and the rapidly advancing technology of lithium-ion batteries. Over the next five years, Tesla’s new “gigafactory” in the USA is expected to double the world’s lithium-ion battery supply for the stationary energy storage market, a vital enabling technology at the dawn of the upcoming solar age.
Off-grid electrification of the global rural community should catalyse the economic and social development of marginalised people. A number of available technologies as well as new opportunities on the near horizon are considered to be viable and environmentally sound, and will contribute to local economic development.
Author
Dr R. Vasant Kumar of the Department of Materials Science, University of Cambridge, has been conducting world-leading research in materials chemistry reactions at the cutting edge of new applications within an ecological calculus.